Many of the application technologies used in specialty crop production today are based on axial fan airblast sprayers (Figure 1). Airblast sprayers were first developed in the 1950s when orchard trees were 6 meters tall or more; today 2-4 meter tall trees are typical. Airblast sprayers are versatile, reliable, and can be modified to fit numerous types of crops, all of which are reasons for their continued popularity. Despite their popularity, air-blast sprayers have long had a reputation for inefficient application characteristics. Sensor controlled spray systems were first designed in the 1980s as a way to reduce labor costs and pesticide waste. Sensor controlled spray systems are receiving renewed interest as their reliability has improved and more options have become available.
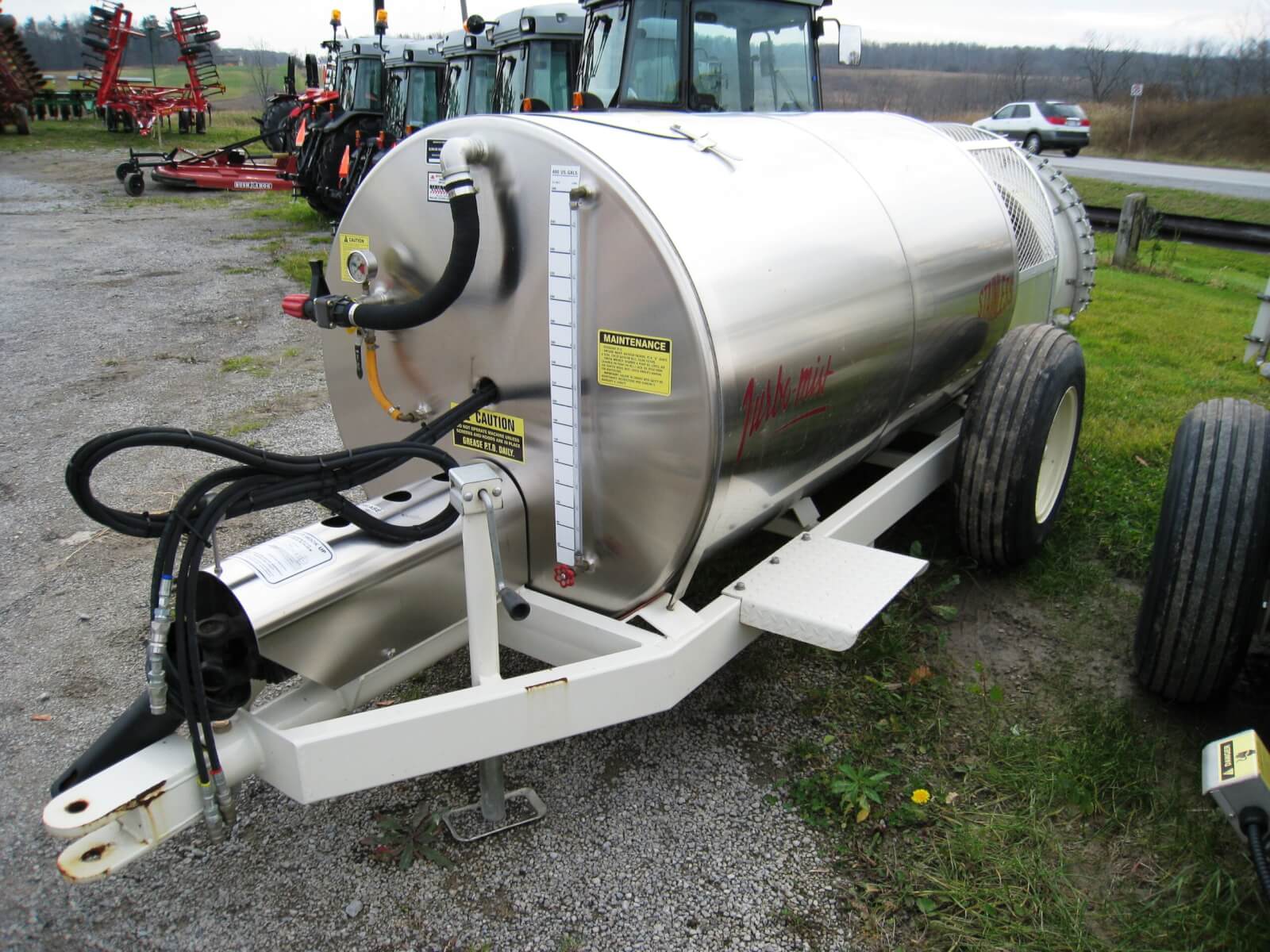
Sensor Sprayer Types
There are two main types of sensor sprayers:
A. On/off sensor sprayers operate by using the sensor information to automatically turn on individual nozzles or sections of nozzles on the spray boom when plants are sensed. Likewise when no object is in the sensing range of the sprayer, the spray will be turned off. (Figure 2A).
B. Crop adapting sprayers are similar in that the sensor is used to turn the sprayer on and off as it passes by plants, however they go a step further by changing application volume, air volume, air flow direction, or a combination thereof in response to plant characteristics. (Figure 2B).

On/off and canopy adapting sensor sprayers both typically have an override where the user can bypass the sensor system and spray as they would with a standard sprayer if the sensor components of the sprayer are malfunctioning.
Sensors
Crop sensing systems are the eyes of the sprayer and determine crop shape by emitting and/or receiving signals. There are 4 basic sensor types used in sensor sprayers: infrared, ultrasonic, plant fluorescence and LiDAR. Each one of these sensors emit their own specific band of waves aimed at the plants that then bounce off the plants and back to the sensor. After the wave returns to the sensor, the time that the wave took from when it was emitted until when it returned is calculated. This calculated time from when the sensor sends the wave until it bounces back to the sensor is called the time of flight (TOF). The TOF is used to calculate distance and/or plant characteristics which are used to target spray to that area. For some sensor types, multiple sensors are required to resolve plant structure characteristics, while other sensor types require only one.
Infrared (IR) sensors detect IR radiation emitted from plants. Atmospheric conditions such as humidity and temperature have little impact on IR sensing accuracy. However, light intensity, plant and leaf appearance, and driving speed can affect the accuracy of these sensors. Low light conditions, such as during dawn and dusk when red wavelengths of light are more abundant, are known to interfere with IR sensor functioning for around 30 min. During dawn and dusk, IR sprayers could be operated in standard mode via an override on the spray controller. The inability to resolve characteristics of plant structure makes IR sensors suited to less complex applications such as triggering the sprayer on and off at a plant. Even with their limitations, the low cost of IR sensors makes them economically viable for commercial sprayers. IR systems can be used on air-blast sprayers for foliar applications of pesticides to trigger the release of spray when a plant canopy is detected. Additionally, these systems can be used for herbicide sprayers where the sensors are aimed at the trunks of trees/vines and turn off the sprayer as they pass the trunk to avoid direct application to it. Conversely, the sensors can directly spray only the trunks to specifically target suckers.
Unlike the other sensors in this list that all emit electromagnetic waves, ultrasonic sensors emit high-frequency sound waves to measure objects. A sonic emitter generates an ultrasonic sound wave, a sensor detects the returning sound wave, and a chronometer measures the TOF of the wave to gauge distance. This process is like how bats or dolphins use echolocation to navigate and search for food. When arranged in an array, ultrasonic sensors can detect objects with approximately 10 cm resolution, allowing for calculation of canopy volume with similar accuracy to taking manual measurements. Bumps and swaying from rough driving conditions can change the accuracy of the ultrasonic sensor because movement affects the angle of signal detection. The initial patents on ultrasonic sensors expired decades ago, so continued off-patent development has improved their quality and capability while reducing costs. Comparatively, ultrasonic sensors are more expensive than IR sensors but less expensive than laser sensors.
Laser sensors come in wide variety of configurations depending on the application, but those typically used to characterize plants are either 2 dimensional (2D) or 3 dimensional (3D) in the space that they can sense. These sensors are referred to as LiDAR sensors which is an acronym meaning “light detection and ranging.” 2D sensors emit light beams in a 2D plane around the sensor using a mechanical scanner. Picture an arc around a central origin point (See Figure 2B). 3D sensors emit laser beams in a 3D area surrounding the sensor, like the shape of a sphere. In both 2D and 3D laser sensors there is a spinning mirror inside the sensor that reflects the laser in every direction possible within sensor’s field of view, then measures the TOF from the sensor to plants and other objects. Compared to other sensors, LiDAR most accurately measures crop structure. Numerous LiDAR sensors are available for industrial applications such as agriculture.
Sensor Type | Measurement Method | Pros | Cons |
Infrared | Detection of infrared waves emitted or reflected from plants. | Little impact of temperature and humidity on sensing accuracy. Low cost. | Sensing ability impacted by red light intensity and driving speed. Narrow field of view and short sensing distance. Unable to determine plant structure characteristics. |
Ultrasonic | Measurement of the distance to objects using sound waves. Uses time of flight concept. | Ability to determine plant structure characteristics. Relatively easy to implement. | Limited resolution of plant structure. Need multiple sensors to detect plant structure. |
Plant Fluorescence | Detection of near infrared fluorescence from green plant surfaces. | Ability to determine plant structure characteristics. Rapid data acquisition. | Need multiple sensors to detect plant structure. Sensors affected by background lighting, requiring frequent calibration. |
LiDAR | Measurement of the distance to objects using laser beams. Uses time of flight concept. | Rich data acquisition capability. Fine resolution of plant structure. High speed of measurement. | Data acquisition affected by tractor bouncing which requires correction. Delicate moving parts inside sensor. |
Plant fluorescence sensors are another sensor type used in sensor controlled sprayer applications. These sensors emit a wavelength of light in the visible spectrum and detect the fluorescence reflected back at the sensor from the plant. Specifically, these sensors detect near infrared wavelengths of light, which plants reflect readily. These sensors have a spatial resolution of about 10cm2 and can detect a plant area as small as 10mm2 (Genna, Gourlie, & Barroso, 2021). Plant fluorescence sensors can collect plant structure data which can be stored and used for further agricultural planning. These sensors are most widely used on “weed-seeing” herbicide sprayers to detect green weed tissue contrasted with the soil surface, however they are also integrated onto canopy sprayers to trigger the spray when a green canopy is sensed.
One other sensor is needed for sensor controlled canopy sprayers. Ground speed sensors are used in combination with crop sensors to sync spray release to plants as the sprayer moves through the field. Currently, sensor spraying systems are not directly connected to the speedometer on the tractor, so a separate speed sensor is needed to convey the sprayer ground speed to the sensor system. Maintaining accurate speed sensing is critical to ensure spray is released on target. Some spray systems derive speed from tractor wheel bolt sensors or with radar sensors that use Doppler technology. The simplest adjustment of sensor controlled spray release is through mechanical adjustment of the horizontal location of sensors and delay adjustments to set when the sprayer turns on and off after objects are sensed.
Spraying with Sensor Sprayers
Sensor sprayer efficacy and efficiency
Insect pest and disease control with sensor controlled sprayers has been widely shown to be similar to that of standard sprayers. For example using a LiDAR based variable rate sprayer on a diversified fruit farm in Ohio resulted in equivalent control of disease and insect pests such as codling moth, powdery mildew, and scab on apples, oriental fruit moth and brown rot in peach, spotted wing drosophila and mummy berry in blueberry, and anthracnose in black raspberries compared to when a standard air blast sprayer was used (L. Chen, Wallhead, Reding, Horst, & Zhu, 2020). Control was achieved on those crops with 29%, 48%, 52%, and 59% lower spray volume when applying on peaches, blueberries, black raspberries, and apples, respectively (L. Chen et al., 2020). When ultrasonic sensors were used to actuate nozzles in one, three, and seven-year-old apple orchards, apple rust mite (Aculus schlechtendali) and pear psylla (Cacopsylla pyri) were both controlled with similar efficacy to standard sprayers (Koch & Weisser, 2000). Additionally, apple scab (Venturia inaequalis) and apple powdery mildew (Podosphaera leucotricha) were controlled to a similar extent using sensor sprayers as with standard sprayers (Koch & Weisser, 2000; Sedlar et al., 2013). Generally, in crops with a uniform canopy such as vineyards or densely planted orchards, sensor sprayers will result in lower volume savings compared to crops with a more variable canopy.
Operational efficiency improvements
Economic savings from sensor sprayers come from a variety of places. The most direct savings come from a reduction in the cost of spray materials required to treat an area. Pesticide material savings will be most significant when sensor sprayers are used in areas with sparse foliage or irregularly shaped crops. Variability in the size of plants across a field is common in some specialty crop operations. Variability can be due to multiple plant varieties being grown, the death of plants or limbs, and replanting. For example, in almond and citrus groves where sick trees are removed and replaced, causing a mosaic of differently aged trees and sizes of canopies. Another aspect of sensor sprayers that can save time and money is automatic adjustment of nozzles as the plant progresses in growth during the season. Early in the season when there is not much foliage present, a sensor system will automatically adjust which nozzles are on to apply the product to where it is needed. This can save the operator the time of manually adjusting nozzles.
Labor savings from less time spent on a given spray event is another significant source of savings when using a sensor controlled sprayer. For example, a 100 acre orchard getting air-blast sprayed at a target rate of 2 acres per hour. At 60% efficiency (due to fill-ups) the sprayer would cover 1.2 acres per hour, with the whole field being completed in 83 hours of work. By implementing a sensor sprayer, the efficiency could increase to 80%, and the area covered would increase to 1.6 acres/hour. In this scenario, the field could be sprayed in 62 hours, about 20 hours less. If an operator is paid $20 an hour this would result in labor costs per acre of $16.60 for the 60% efficient sprayer and $12.40 for the 80% efficient sprayer. Therefore using the 80% efficient sprayer could result in $420 savings for the farm per application. A larger orchard with more than one sensor sprayer would accumulate savings quicker, due to incremental increases in overall operational efficiency for each sprayer as they are added (Tona, Calcante, & Oberti, 2018).
In addition to monetary savings, driver fatigue is reduced as the number of hours the tractor is in operation goes down. Also, a reduction in the number of sprayer fill-ups required leads to lesser labor and fuel costs, in addition to a lower amount of wear on the tractor. When spray operations can be completed quicker, it can also make it easier to fit sprays into windows of good weather or during critical application windows, such as when a plant pathogen or pest is reproducing.
Environmental benefits of using sensor sprayers
Environmental benefits of using a sensor system include reduced chemical load on the non-target crop environment, beneficial organisms, and workers. Spray drift can be broadly defined as any spray that does not get deposited on the intended target. Drift can be deposited on the ground near the intended target, or can be carried further, eventually landing on the ground or on non-target plants. Ground deposited drift is especially common in gaps between trees, which can result in significant pesticide load on the environment. In California almond orchards, ultrasonic sensor sprayers reduced ground deposition by 72% compared to a standard axial fan air-blast system (Giles et al., 2011). Airborne drift from over application is another significant source of non-target pesticide load from air-blast sprayers. In apple orchards, 23-45% of the applied pesticide volume has been observed to drift off target (Pergher, Gubiani, & Tonetto, 1997). Canopy adapting sprayers can be particularly effective at reducing spray drift. A study looking at three different canopy stages in an apple orchard from early to late season showed reductions in spray drift of 70-100% using a canopy adapting sprayer (Y. Chen, Zhu, Ozkan, Derksen, & Krause, 2013). Lower non-target chemical loads also help decrease the rate of development of pesticide resistance because less pesticide residue is present on non-target locations (Verweij, Snelders, Kema, Mellado, & Melchers, 2009). Other considerations include less pesticide contamination of surface and groundwater and lower chances of exposure on non-target organisms such as beneficial insect populations and livestock (Pimentel, 1995). These benefits incrementally improve the vitality of the agricultural landscape, and should not be overlooked when thinking of implementing a sensor sprayer.
One step further: autonomous sensor control airblast sprayers
Sensor controlled sprayers help to increase spray application efficiency a lot, but there still needs to be an educated applicator driving the tractor and operating the sprayer. Agricultural labor availability has become increasingly less reliable and more expensive, so some sprayer manufacturers are producing autonomous sprayers that can be monitored remotely during a spray process. The GUSS sprayer (Global Unmanned Spray Systems) is fully autonomous self-propelled sprayer. GUSS sprayers come in a large size designed for mature orchard trees and a mini GUSS that is designed for grapes and small fruits. The GUSS systems can be manufactured with or without ultrasonic sensors. Up to eight GUSS machines can be running at the same time while being monitored by one individual. Another autonomous system is the result of a collaboration between Hol Spraying Systems (HSS) and Agxeed, called the Agxeed HSS (Figure 3). This is a multi-purpose agricultural robot with a standard 3 point hitch. A 2000L tank can be integrated with the machine to supply the sprayer with water. The sprayer used on the robot can be integrated with HSS’s Intelligent Spray Application system to modulate spray output as plants are sensed. This system uses plant fluorescence sensors and mapping software to apply pesticides with variable rates while also taking plant structure data that can be used for other purposes. In addition to the sprayer, standard agricultural implements such as mowers or grinders can be hooked up to the robot for autonomous operation. Another high tech autonomous sprayer is the Jacto Arbus 4000 JAV. This is a self-propelled sprayer that is equipped with sensors to modulate spray volume in real time in addition to a separate electronic driveline to actively modulate air volume in real time to match the target canopy. All three of these autonomous sprayers offer lower labor requirements and more efficiency than manned-sprayer applications.

Conclusion
Sensor sprayers are another great tool available to specialty crop growers to improve spray application efficiency. The range of sensor types available are suited to a wide variety of crops and applications, and in most cases can be added retroactively to growers current sprayers. The benefits of sensor controlled spray systems are many, from economic to environmental, and all work to improve the vitality of the agricultural landscape.
This article was adapted from Pacific Northwest Extension publication #727 (Warneke, Pscheidt, Rosetta, & Nackley, 2019). Freely available online from Oregon State University, Washington State University, or University of Idaho extension.
This work was provided in part by the USDA-ARS Integration of Intelligent Spray Technology into IPM Programs in Specialty Crop Production (Project Number 58-5082-2-010).