Originally published in: Wolf, T.M. and Downer, R.A. ILASS Americas, 11th Annual Conference on Liquid Atomization and Spray Systems, Sacramento, CA, May, 1998
Note to reader: It’s been nearly 23 years since we wrote this paper at the invitation of organizers of the Institute for Liquid Atomization and Spray Systems Conference. At the time, custom operators, not farmers, bought self-propelled sprayers. Air-induction tips had just been introduced. Pulse-Width Modulation was only beginning to be available. GMO crops were available but not widely adopted. Buffer Zones were more rumour than policy. How badly out of date are the thoughts we mulled over?
Abstract
The goals of an agricultural spray application are to provide effective control of the pest at low cost without adverse environmental impact. A spray must transport effectively from the atomizer to the target, be intercepted and retained by the target, and form a biologically active deposit. Improvements in efficiency are elusive because of interactions between successive stages in dose transfer. Progress will depend on atomizers providing increased control over droplet size and velocity spectra without sacrificing mechanical simplicity: (a) elimination of the interdependence of flow rate and spray quality, (b) control over size span at any given nominal diameter, (c) reversal of present relationship between droplet size and velocity. Such an atomizer would drive a new research thrust to improve spray efficiency
Introduction
Polydisperse sprays provide consistent results yet suffer from inherent inefficiencies in dose transfer. Drift potential and poor spray retention at the extreme ends of their spectra are classic examples of this inefficiency, and environmental aspects of spray application have been criticized as a result (Pimmentel and Levitan, 1986). Yet, despite ongoing research, efficiency breakthroughs remain elusive (Hislop, 1993). Due to the interdependency of the factors governing dose transfer, progress in one area (i.e., greater retention with finer sprays) has often been at the expense of spray drift, and vice versa (Young, 1986). Theoretical improvements in efficiency with monodisperse sprays (Controlled Droplet Application, CDA) have not translated into widespread adoption due to drawbacks in consistency and robustness of the results. After 50 years of research, the same compromises which have been discussed since the early days of spray application are apparently still unresolved.
Nozzle designs have certainly improved – wider pressure ranges, improved spray patterns, more options for achieving various spray qualities, better quality, longer wearing materials, and lower costs are all important for the end-user. But the basic atomizer – the hydraulic flat fan nozzle generating a polydisperse spray – has hardly changed over the years.
A New Start?
The questions posed in this paper are: If a biologist could design the ideal spray, what would it be? What are the criteria for achieving the best result in the most efficient manner? Such a discussion represents a unique opportunity to think about what we know about sprays and their biological impact, providing atomizer design information to meet our future needs.
Unfortunately, biologists still know relatively little about the impact of kind of spray quality on efficacy. General statements can be made relating spray quality to herbicide, insecticide, or fungicide effectiveness, but for the most part, the ideal spray or subsequent deposit has still not been defined for most situations (Hislop, 1987). The situation reflects the lack of choice in spray atomization, creating a catch-22: not being able to easily produce customized sprays has made it difficult for biologists to identify (without confounding factors) the ideal spray for any particular situation. Further, the need by the industry for a simple, reliable, and standard application system has inherently hindered efforts to optimize the system. All stakeholders will need to be flexible to present a fertile environment for improvements to take hold.
“Integrated Spray Management”
In this era of integrated pest management, cropping systems are optimized to provide the most effective pest management strategy on a case-by-case basis with minimal crop protection agent (CPA) use. This underlying philosophy can be extended to spray application. When CPAs are used in such systems, they, too, must rely on diverse strategies to make them more efficient. Within this philosophy, a single standard application technique for all pests will not be acceptable. Two developments are needed to put such a development into action: (a) an application system capable of delivering a wide variety of spray qualities (droplet sizes, spans, velocities) at a range of carrier volumes; and (b) the knowledge to utilize specific spray qualities under identifiable conditions.
Application Objectives
During the development of such a new application philosophy, the objectives for spray application must remain clear. They are to deliver a CPA in its most effective form to the pest, with no off-target effects, at the lowest possible cost, i.e., effective, economical, and environmental.
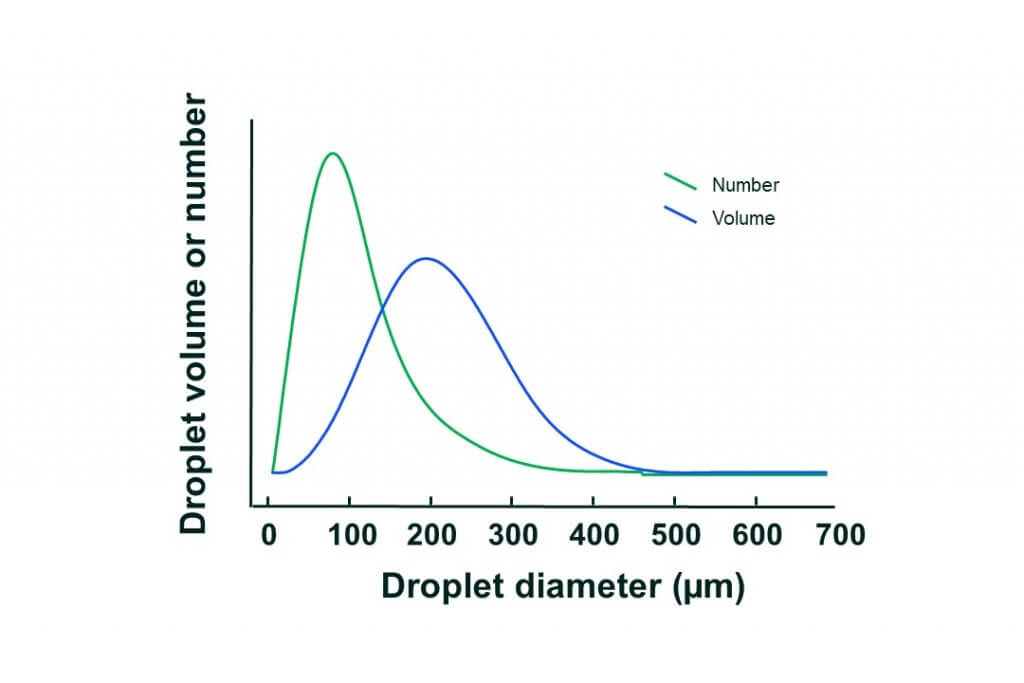
Figure 1: Typical droplet number and volume spectra for an agricultural hydraulic flat fan nozzle
The status quo for most post-emergent CPA applications is the hydraulic flat fan nozzle. As we know, such a nozzle produces a heterogeneous mix of fine and coarse droplets (Figure 1) with a droplet speed and size relationship (Figure 2). This nozzle has frequently been criticized for inefficiency because only a small portion of spray is optimally targeted (Adams et al., 1990). At the same time, it has been applauded for consistency because a portion of its size and velocity spectrum (although not necessarily the same one) is usually appropriate for the pest complex at hand.

Figure 2: Typical velocity spectrum for an agricultural hydraulic flat fan nozzle.
The most frequently documented drawbacks of the hydraulic nozzle are driftability of fine, and poor retention of coarse components. An additional drawback is interdependence of flow rate and droplet size for any given nozzle, i.e., at the same work rate, lower carrier volumes are applied with finer sprays. Research into droplet size effects has been difficult because no variable can be held constant while another changes. Keeping dose constant, studies of carrier volume have to accept a simultaneous change in travel speed or droplet size, droplet size studies have to contend with changes in droplet density, and droplet density studies must alter active ingredient concentration. Given the complexity of the problem, few researchers commit themselves to solving these dilemmas.
Maximizing Effectiveness – No Easy Answers
For any given spray mixture, an atomizer controls spray pattern, droplet size, and droplet velocity. Spray patterns determine spatial uniformity. Droplet size and velocity in turn affect spray fate by controlling canopy penetration spray interception, spray retention, spray coverage, evaporation rate, etc. Considering the variety of active ingredients, formulations, concentrations, environments, pests, and plant canopies present, it is not surprising that the scientific body of evidence is often contradictory (Knoche, 1994). It should also come as no surprise that there is no single “best” droplet size to optimize these factors.
Basic principles: In order to better understand why a single ideal spray cannot exist, a brief review of the principles of spray drift, interception, and retention are appropriate. Larger droplets are driven mostly by inertial and gravitational forces (Spillman, 1984). As such, they tend to have vertical trajectories from which they cannot easily be displaced. This makes them a good choice for drift reduction, and also for canopy penetration into vertically oriented canopies, such as cereal grains. Collection efficiency by a target is a function of target size and orientation – horizontally oriented, larger objects will be favoured by larger droplets. Spray retention is a function of leaf surface wettability and microstructure, as more difficult to wet species will be more likely to reflect larger droplets (Hartley and Brunskill, 1958).
Small droplets, on the other hand, are more subject to viscous drag, have shorter stop distances, and can therefore move with local air turbulence to reach shadow regions (Nordbo, 1992). Thus the finer sprays have a propensity for displacement from their flight path by air turbulence, but they also are better able to penetrate dense broadleaf canopies because they can move around larger objects. Small, vertically oriented objects such as stems and petioles have the best collection efficiency for small droplets.
Upon depositing successfully on a target, the deposit must be in a form which exerts the desired biological effect. Given the same spread factor, deposits with greater volumes remain wet longer, providing more opportunities for uptake into the leaf. Small droplets provide more efficient coverage per unit volume, but dry rapidly, which may limit their uptake.
Uptake and translocation of active ingredients by biological targets are physical processes driven by concentration gradients. Concentrations of active ingredients and surfactants per unit leaf area are a function of carrier volume, droplet size, and spread factor. Less than optimum concentrations can result in reduced uptake and translocation (Wolf et al., 1992).
Further complications arise due to the heterogeneous nature of weeds. Individual regions of weed plants have unique anatomical and physiological features that can affect retention, uptake, and translocation processes on a spatial level. For example, Merritt (1982) showed that for wild oats (Avena fatua), younger leaves and the basal region of leaves absorbed more difenzoquat than older leaves.
All these factors conspire to complicate the quest for optimization in a field setting.
The Ideal Spray
Based on the previous discussion, it may be obvious that a single droplet size cannot meet all demands within such a complex system. Therefore our focus must shift from a theoretical optimum solution, as was the basis for controlled droplet applicators (Bals, 1980) to one which emphasizes flexibility.
One advantage of speaking on behalf of biologists is that one can feign complete ignorance about atomization, and propose seemingly ludicrous ideas. Perhaps a prerequisite to a fresh approach is such ignorance.
Biologists need a spray to not only implement optimum application, but as a means with which to learn how to optimize the process in the first place. Further, since there is no single optimum spray quality to meet all application scenarios, the most important feature in a spray is flexibility. The following features will be important:
Spray quality independence: The first criteria is the ability to adjust spray quality easily, without affecting carrier volume or droplet velocity, and vice versa (Figure 3). A shift towards a coarser or finer spray can then be achieved without introducing other confounding effects. Some progress has already been made in this area (Giles and Comino, 1990).

Relative span factor flexibility: The relative span factor of the spray should be adjustable (Figure 4). It will be important to narrow the broad spectrum sprays produced by flat fan nozzles to determine the importance of specific droplet sizes. While such research was conducted during the 1970s and 1980s with controlled droplet applicators (CDAs), the unique droplet velocity associated with such atomizers would question results if they were to be applied using hydraulic atomizers.

Velocity control: The third criteria is for improved droplet velocity control. The droplet velocity dependency on size has meant that in the absence of air assist, smaller droplets are always moving slower. This factor has reduced the efficiency of their collection and made them more drift prone. Additionally, the larger droplets, being faster moving, were more likely to rebound from targets. Acceleration of small droplets is a strategy for reducing spray drift and enhancing collection efficiency, but greater velocity for larger droplets may reduce the efficiency of their retention by the target. If the droplet size – velocity relationship were reversed, then smaller droplets would be less drift prone and larger droplets would be less likely to rebound (Figure 5).

Spray heterogeneity: Spray heterogeneity will remain important in an optimized system, especially in the absence of specific knowledge on droplet function by size class. In this sense, a polydisperse spray does more than provide insurance for changing conditions, it adds diversity to static conditions which strengthens the overall effect. While a quantitative dose-based approach to CPA delivery is often appropriate, it under-emphasizes the role of deposit structure and spray redistribution, where quality is more important than quantity (Wolf, 1996). For example, let us assume that canopy penetration is maximized with a spray of 400 µm VMD, with a relative span factor of 0.7. In such a spray, fine droplets contribute relatively little to overall dose. However, their ability to redistribute in the canopy, targeting areas left untouched by the larger droplets may be more important than their total dose contribution would suggest. In this way, they provide benefits which are total dose independent. A heterogeneous spray would ensure that these benefits remain.
Deposit uniformity: Efforts at optimizing dose transfer are compromised if spatial dose uniformity cannot be maintained within the treated area. High deposit variability has been associated with reduced control of insects (Uk and Courshee, 1982; Cooke et al., 1986). As such, uniformity remains a fundamental requirement for spray application and should not be compromised with new atomizer designs.
Environment as a Priority
Spray must land on the intended target, be it a plant, insect or ground, and in some cases on the optimal pest part, i.e., specific leaves, leaf sides, stems, etc. Off-target placement not only represents inefficiency, but also undesired environmental input. With any application system, an important criteria is the ability to manage off-target impacts.
Past solutions to spray drift or droplet rebound has been two-fold: (a) eliminate those droplets which do not impact on the target efficiently, or (b) protect them from displacement. For spray drift, the elimination of small drops through production of coarse sprays has been successful (Edwards and Ripper, 1953). The challenge is to provide drift protection without compromising the advances made in the previous exercise of maximizing effectiveness. The protection of fines with barrier (shrouds) is an effective strategy for reducing drift, and provides the advantage of maintaining a spray quality established to meet separate criteria (Wolf et al., 1993). Another successful strategy has been to assist transport of fines with an external energy source (air or electrostatics). This also allows the preservation of an optimized spray quality, with the added advantage of modifying the droplet velocity spectrum in favour of canopy penetration.
Nozzle design may offer some opportunities for the reduction of rebound. Novel atomization systems such as venturi or twin-fluid nozzles, which offer air-inclusion in droplets, may reduce rebound of larger droplets. If larger droplets are required, but retention is of concern, such approaches may be useful. Spray adjuvants can also play important roles in this area (Downer et al., 1995)
Economical Considerations
Underlying any attempt to provide effective pest management is an economical consideration. The producer must see a benefit in making a technical investment. Any atomizer solution must therefore not only meet the technical requirements for optimizing dose transfer, it must also be a cost-effective and practical system. A system which is complicated to use is not likely to be widely adopted. Without strategies for implementation by the end user, innovations in delivery are merely theoretical exercises.
Putting it into Practice
During a typical work day, an applicator may be called to treat crops for a range of pests with broad-spectrum products. These pests will likely be present in a range of densities, some above and others below an economic threshold. There may also be a range of canopies present, some broadleaves, others grasses, some dense, others sparse. Depending on the area, there may have been a range of environments under which pests became established, or during which application is made. Each field will also have a range of bordering ecosystems with unique trespass sensitivities.
There will obviously be a limit to the degree of customization that is possible. But some efforts will be rewarded. The applicator uses GPS technology to collect or recall relevant data – sensitive areas, high and low infestation levels, or changes in canopy structure. With the new atomizer, the applicator can emit the most effective droplet size, velocity, span, and dose appropriate for the pest or canopy on a site-specific basis. The use of spray quality classification systems such as those developed by the BCPC and ASAE will guide optimization efforts, but in the end, these classification systems will be too broad to fine-tune the system. A higher resolution, multi-parameter scheme which is sensitive enough to represent the criteria laid out in this paper will be necessary.
Possible difficulties emerge when the system resists optimization. For example, it would be comparatively easy for the applicator to control a broadleaf weed in a grassy canopy, as the size spectrum which optimizes grass canopy penetration is also likely to target the broadleaf weed effectively. If a tank mix is used to control both grassy and broadleaf weeds in this canopy, the applicator now needs a more heterogeneous spray, where a finer component targets the grassy weed, and the coarser component still effectively transfers dose to the broadleaf weed. As the situation increases in complexity, the simultaneous optimization of several criteria will be increasingly difficult.
Conclusions
Only an integrated approach involving all stakeholders (engineers, chemists, biologists, etc.) can result in improved application of CPAs. Individual goals and concerns must be communicated and reconciled in new design efforts. This paper represents a wish list from biologists’ perspectives. While greater flexibility and control are important objectives in our opinion, consideration must also be given to mechanical complexity and cost, possible interactions with formulations exhibiting a range of physico-chemical properties, biocontrol agents, and practical strategies for adoption. A continued willingness to establish and maintain lines of communication and cooperation between these disciplines will be pivotal to success.
Acknowledgments
The invitation by the ILASS Program Organizing Committee to make this presentation is gratefully acknowledged.
Citations
- Adams, A.J., Chapple, A. C., and Hall, F.R.. 1990. Agricultural sprays: lessons and implications of drop size spectra and biological effects. In: L.E. Bode, J.L. Hazen, and D.G. Chasin (eds.) Pesticide Formulations and Application Systems, ASTM STP 1078. American Society for Testing and Materials, pp. 156-169
- Bals, E. J. 1978. The reasons for C.D.A. (Controlled Drop Application). Proceedings of the 1978 British Crop Protection Conference – Weeds, pp 659-666.
- Downer, R.A., Wolf, T.M., Chapple, A.C., Hall, F.R., and Hazen, J.L. 1995. Characterizing the impact of drift management adjuvants on the dose transfer process. In: R.E. Gaskin (ed.) Fourth International Symposium on Adjuvants for Agrochemicals. New Zealand Forest Research Institute, Rotorua, NZ, pp. 138-143.
- Edwards, C.J. and Ripper, W.E. 1953. Droplet size, rates of application and the avoidance of spray drift. Proceedings of the 1953 British Weed Control Conference, pp. 348-371.
- Giles, D.K., and Comino, J.A. 1990. Droplet size and spray pattern characteristics of an electronic flow controller for spray nozzles. J. Agric. Engng. Res. 47:249-267.
- Hartley, G.S. and Brunskill, R.T. 1958. Reflection of water drops from surfaces. In: J. F. Danielli, K. G. A. Parkhurst, and A. C. Giddiford, eds., Surface Phenomena in Chemistry and Biology, Pergannon Press, London, pp. 214-223.
- Hislop, E.C. 1993. Application technology for crop protection: an introduction. Pages 3-12 In: G.A. Matthews and E. C. Hislop (eds.) Application Technology for Crop Protection. CAB International, Wallingford, UK.
- Hislop, E. C. 1987. Can we define and achieve optimum pesticide deposits? Aspects Appl. Biol. 14:153-172.
- Knoche, M. 1994. Effect of droplet size and carrier volume on performance of foliage-applied herbicides. Crop Prot. 13:163-178.
- Merritt, C.R. 1982. The influence of form of deposit on the phytotoxicity of difenzoquat applied as individual drops to Avena fatua. Ann. Appl. Biol. 101:517-525.
- Nordbo, E. 1992. Effects of nozzle size, travel speed and air assistance on deposition on artificial vertical and horizontal targets in laboratory experiments. Crop Prot. 11:272-278.
- Pimentel, D. and Levitan, L. 1986. Pesticides: Amounts applied and amounts reaching pests. BioScience 36:86-91.
- Spillman, J.J. 1984. Spray impaction, retention and adhesion: an introduction to basic characteristics. Pestic. Sci. 15:97-106.
- Wolf, T.M. 1996. Spray application into standing stubble – an exploration of physical and physiological components. Ph.D. Dissertation, Department of Agronomy, The Ohio State University, 192 pp.
- Wolf, T.M., Grover, R., Wallace, K., Shewchuk, S.R., and Maybank, J. 1993. Effect of protective shields on drift and deposition characteristics of field sprayers. Can. J. Plant Sci. 73:1261-1273.
- Wolf, T.M., Caldwell, B.C. McIntyre, G.I., and Hsiao, A.I. 1992. Effect of droplet size and herbicide concentration on absorption and translocation of 14C‑2,4‑D in oriental mustard (Sysimbrium orientale). Weed Sci. 40:568-575.